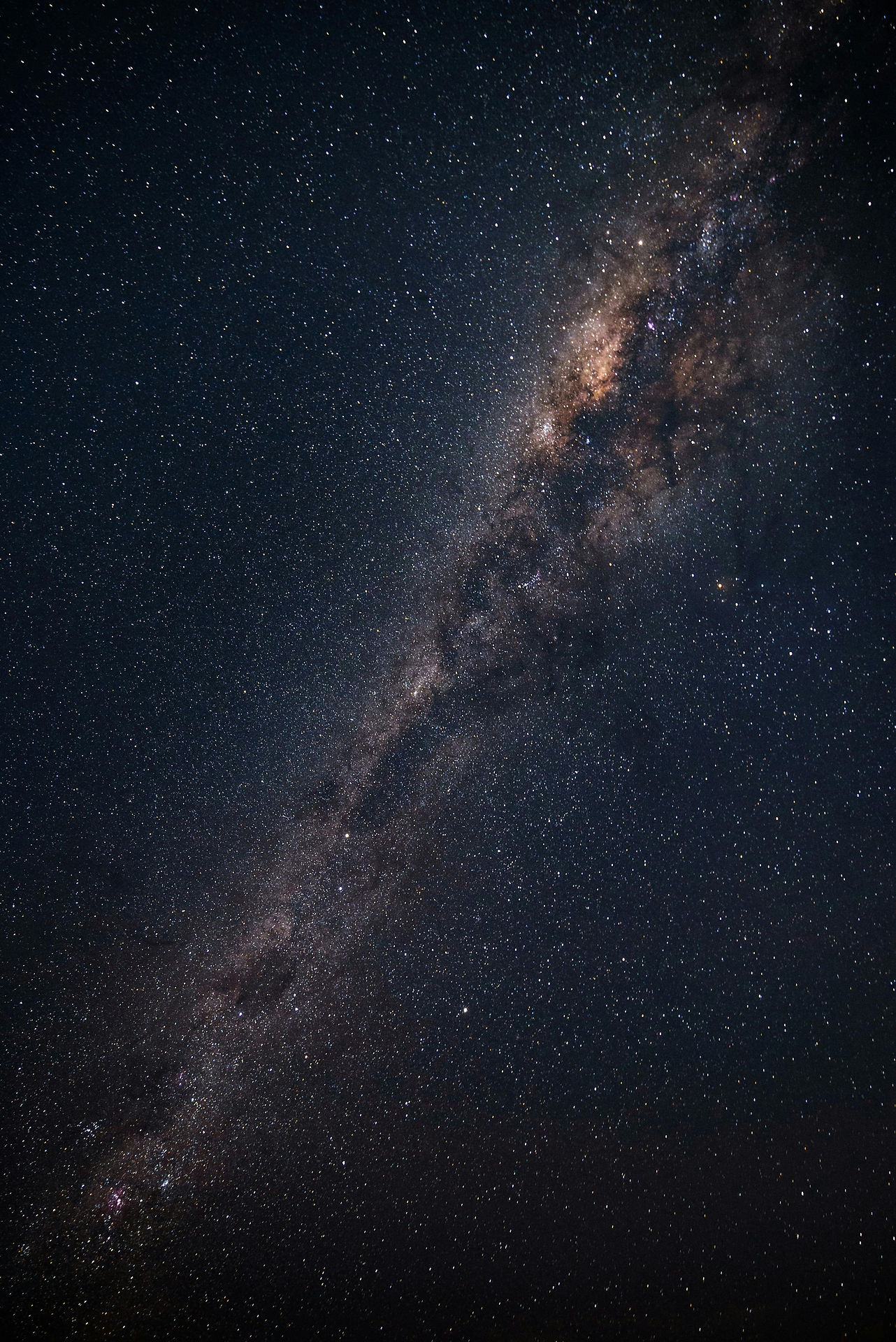

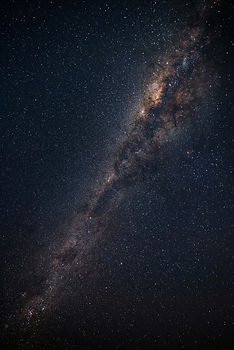
Space Travel
Space travel has been a fascination of science fiction filmmakers and audiences alike for decades. From the early days of black-and-white sci-fi to modern cinematic masterpieces, the depiction of space travel has evolved dramatically, becoming more grounded in real scientific principles. Two of the most intriguing concepts often explored in these films are artificial gravity and gravitational slingshotting. But how realistic are these depictions?
​
On this page we will explore Interstellar and 2001: A Space Odyssey and their attempts to portray space travel with a level of scientific accuracy with a focus on these two crucial aspects of space travel assessing their scientific accuracy while acknowledging the necessary Hollywood embellishments that make for thrilling storytelling. We aim to separate fact from fiction and appreciate the balance between entertainment and realism in cinematic space travel.
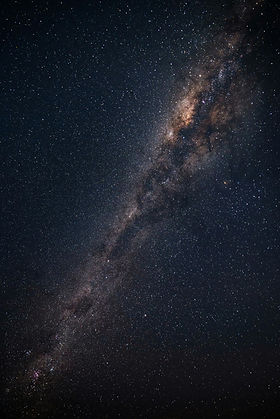
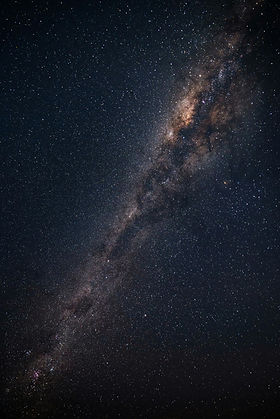
Key Sections
In this section, we provide all the necessary background information to understand the concepts of artificial gravity and the slingshot manoeuvre.
In this section, we evaluate Interstellar's and 2001: A Space Odyssey's portrayal of artificial gravity and the slingshot maneuver.
In this section, we critically compare the portrayals of the two movies.
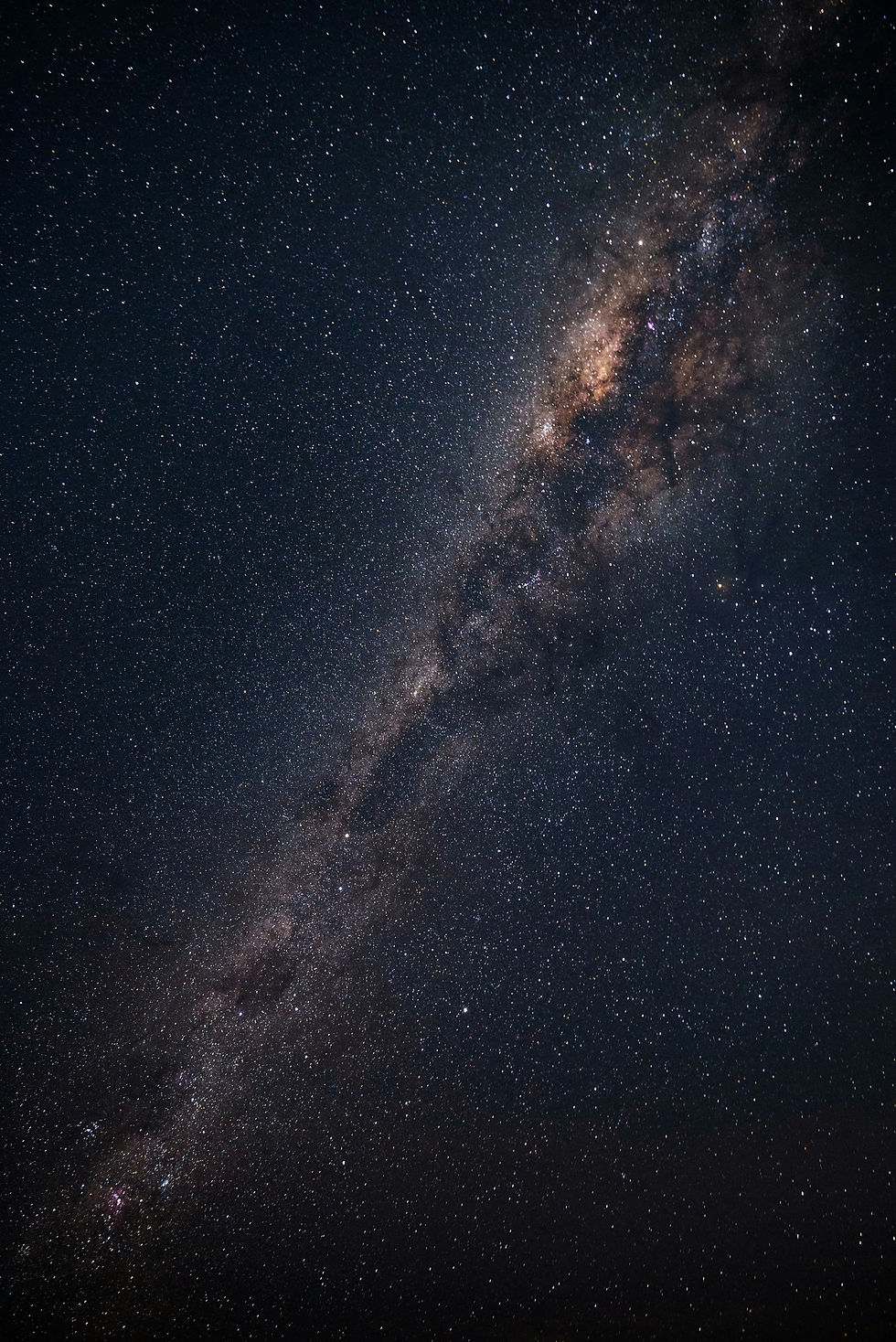
Background
Theory
Fairground ride exhibiting the centrifugal effect from fast rotation, which causes visitors to be held against the walls.
​
Image sourced here.
“Artificial gravity” is exactly as you’d imagine – it is a man-made force designed to mimic Earth’s gravity in environments where it is absent, such as in space. Dissimilar to real gravity, which is caused by the mass (weight) of a planet, artificial gravity is simulated via circular motion, specifically rotation. It exhibits a particular utility for astronauts in zero-gravity space for long periods of time, such as preventing them from floating out of bed during the night (uncomfortable, I imagine).
​
​
​
​
​
​
​
​
​
​
​
​
​
​
​
​​​
​​​
​Numerous factors contribute to the strength of this artificial gravity, such as the radius of the rotating spacecraft and the speed of rotation of the spacecraft - the larger the radius and/or rotation speed, the stronger the artificial gravity produced. See our interactive “Artificial Gravity” tool - play around with the radius and rotation speed of a spacecraft to see how gravity changes! Numerous challenges arise when considering these factors, however.
​
Due to the radius of the spacecraft being proportional to the strength, a spacecraft of small radius must rotate at very high speeds; this can result in discomfort. Further to this, a spacecraft with a radius not that much larger than a person would result in the astronaut experiencing significant variations in gravity in their feet and head. Since their feet are much further from the centre of rotation than their head, their feet will experience a much greater gravity than their head. Another challenge associated with artificial gravity is the “Coriolis effect”; this is an unexpected sideways force associated with the movement of an astronaut either toward or away from the centre of rotation. It can affect the semi-circular canals of the ears and result in nausea and dizziness.
​
Gravity assist, also known as a gravitational slingshot, is a technique used in space travel to change the speed and path of a spacecraft by using the gravity of a planet or other celestial body to pull the spacecraft along with it. The concept of gravity assist is deeply rooted in Newtonian mechanics.
​
From Newton’s first law, an object in motion will remain in motion at a constant velocity unless acted upon by an external force. In space, this means a spacecraft will continue in a straight line at constant speed unless it encounters another force. Also, from Newton’s third law, any gain or loss of kinetic energy and linear momentum from the spacecraft must be lost or gained by the object it uses for the assist. The objects used are usually planets but Interstellar explores the concept of using a black hole for this, which we will get more into later. With all cases of gravity assists used, the object is so much larger than the spacecraft that its change in velocity is minute in comparison.
​
The key point for gravity assist’s success is from the motion of the object used for the assist. For example, using Jupiter as a gravity assist to speed up requires it to be moving in the same direction as the spacecraft relative to the Sun. Viewed from the reference frame of Jupiter the spacecraft’s overall speed change may be zero, but viewed from the Sun it increases. However, if the spacecraft were to fly in the opposite direction to the planet’s motion, it could potentially slow down relative to the Sun. The manoeuvre needs to be carefully planned so that the spacecraft can interact with the planet’s gravity at just the right angle and velocity to maximize the boost in speed and trajectory. Our Interactive tool shows how the initial dynamics affect the success of gravity-assists.​​
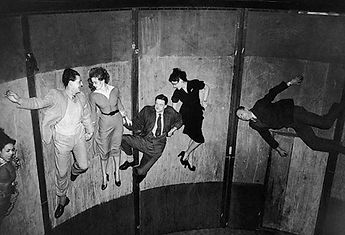
In a rotating spacecraft, the floor provides a “centripetal force”, which acts towards the centre of the rotating body; this force keeps the astronaut rotating in a circular motion. Intuitively, however, this does not make sense – a force acting towards the centre of the spacecraft would see that astronauts fly towards the centre of the spacecraft, yet they stay planted; this is a consequence of Newton’s 3rd law. Instead, the so-called “gravity” that the astronauts experience is attributed to an outward-acting “centrifugal” force, equal and opposite to the centripetal force, and this “pushes” them to the floor.
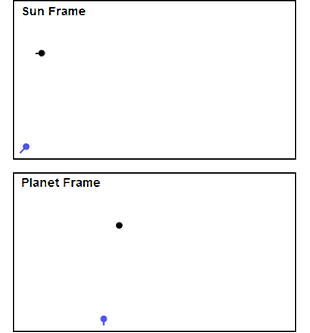
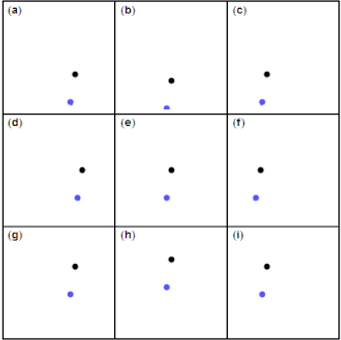
GIFs demonstrating the dynamics of gravity assists. How the same maneuver may be seen in different reference frames (left) and how different initial conditions can affect the outcome of the assist (right). GIFs courtesy of The Planetary Society. See our interactive tool to experiment with the dynamics more.
History
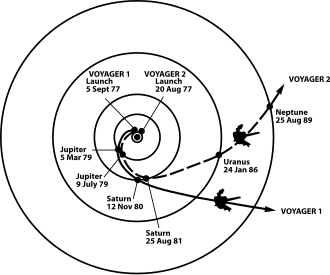
Trajectories of the two voyager probes on their journeys, showing dates of close flybys of gas giants giving gravity assists.
​
Image created by NASA.
The concept of artificial gravity dates back to the early 20th century, many years before humans ventured to space. Some of the earliest proposals for artificial gravity were inspired by amusement park rides that demonstrated the effects of centrifugal force. One of the first famous artificial gravity concepts originated from Konstantin Tsiolkovsky, a Russian rocket scientist. He envisioned a rotating space station with artificial gravity produced via angular motion, i.e. rotation.
​
By the mid-20th century, the effects of weightlessness (or microgravity) in space were being recognised; these included muscle atrophy and a decrease in bone density, both suboptimal symptoms for astronauts to endure for prolonged periods in space. NASA and other agencies investigated various models in an effort to combat these effects, including tethered spacecraft systems.
​
Despite investigation and theoretical feasibility, however, artificial gravity has rarely been tested in actual space missions; the most notable attempt was of the Gemini 11 mission in 1966. The spacecraft was connected by a 36-meter tether to the Agena target vehicle, and, by firing side thrusters, the system was made to rotate slowly. As expected, artificial gravity was produced, though only to a small degree: 0.00015g. Despite the effects being far too weak to be felt by the astronauts, objects were observed to move towards the “floor” of the capsule. A success!
​
Other larger-scale models were proposed, such as the Von Braun Wheel (1952) resembling what was later depicted in the movie 2001: A Space Odyssey; The Stanford Torus (1975). Due to the immense costs associated with engineering these spacecraft and the limitations of technology at the time, these models were never constructed.
​​​
​​
​
​
​
​
​
​
​
​
​
​
​
​
​
​
​
​
​​Arguably the most famous missions that used gravity assist were from the Voyager programme. Voyager 1 is currently the most distant human-made object from Earth which as of the February 2025 is over 25 billion kilometres away from Earth. Live updates of both the Voyager’s positions can be found here. To achieve their distant travel Voyager 1 and 2 both used Jupiter’s gravitational pull, with Voyager 1 taking its closest pass to the planet on March 5, 1979, at about 348890 kilometres above Jupiter’s centre of mass. Both space probes successfully carried out their gravitational assist trajectories and headed towards Saturn. Once reaching Saturn, both probes were able to make observations of the planet's surface and its moons. After visiting Saturn, the two probes parted ways forever, Voyager 2 on its travel to Uranus and Neptune, and Voyager 1 on its escape of our solar system.
Gravity assist has been fundamental for exploration of our solar system, being implemented in several space missions. It is typically used as a method to save propellant and reduce expense, allowing for more complex missions to distant planets, moons, or even other star systems. The theory behind gravity assist was first formally proposed in the 1960s by NASA's Jet Propulsion Laboratory. However, its first successful use was in 1959 by the Soviet probe Luna 3 in its mission to photograph the far side of the moon. The satellite’s speed did not change but its orbit was altered enough to allow successful transmission of its data.
Mosaics formed from the photographs taken by the Voyager probes as they made their close passes to the gas giants. (1) Jupiter's Great Red Spot, (2) Jupiter's moon Io, (3) Saturn, (4) Saturn's moon Enceladus, (5) Uranus, (6) Neptune. See the full gallery on NASA's website.
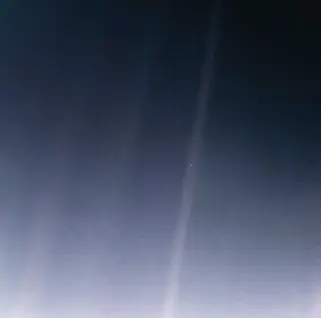

This is Earth!
The 'Pale Blue Dot' photograph our solar system taken by Voyager 1, 23 years after its launch.


Mathematical Appendix
1. Centripetal Force
The force that keeps an object moving in a circular path by acting towards the centre of rotation. It is always directed perpendicular to the object's velocity and prevents it from moving in a straight line due to inertia.
​
​
​
​​
2. Centre of rotation
The fixed point around which an object rotates. This point does not move while the rest of the object follows a circular path around it. In rigid bodies, all points move with the same angular velocity (eg same rpm) around this centre.
​​
3. Newton’s First Law
An object will remain at rest or move in a straight line at constant speed unless acted upon by an external force. This law implies that if no net force acts on an object, its velocity (whether zero or constant) will not change. In other words, objects resist changes in their state of motion.
​​
4. Newton’s Second Law
The acceleration of an object depends on the net force acting upon it and the mass of the object. This law quantitatively relates force, mass, and acceleration, meaning that a larger force is needed to accelerate a more massive object, and the same force will produce a larger acceleration in a less massive object.
​​
​​
​​
5. Newton’s Third Law
For every action, there is an equal and opposite reaction. This means that whenever one object exerts a force on a second object, the second object exerts a force of equal magnitude but in the opposite direction on the first object.
​​
6. Momentum Conservation
The total momentum of a closed system remains constant if no external forces act on it.
​​
​​​
7. Gravitational Well
A conceptual model representing the gravitational potential around a massive object. It describes how objects with mass create a "dip" in spacetime, pulling other objects toward them. The deeper the well, the stronger the gravitational pull. More massive objects create deeper and steeper wells. Objects must gain enough energy (escape velocity) to leave the well.
​​
8. Gravity Assist Conservation of Energy
From the conservation of energy comes the following expression,
​
​
​​​
​​

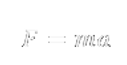
Where Fc is the force on the object, m is its mass, v is its velocity, and r is the radius of the circular orbit.
​
​
Where F is the force exerted on the object, m is the mass of the object and a is the acceleration experienced by the object.
Where Vf and Vi are the initial and final velocities and M is the mass of the first object, vf and vi are the initial and final velocities and m is the mass of the second object.
​
​
​
​
​
​
​
​
​
​
​Where ui and uf are the spacecraft's distant-approach and distant departure velocities relative to the planet.
​

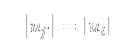
9. Gravity Assist Kinematics
The following vector triangles from the paper by R. C. Johnson show the effects for a flyby where acceleration (left) and deceleration (right) is achieved. The triangle’s common bases are the assisting object’s (eg the planet’s) velocity. vi and vf show the initial and final velocities of the spacecraft from an outside observer. The dashed lines show the spacecraft's initial and final velocities from the planet’s frame.
​
​
​
​
​
​
​
​
​
​
​
​
​
​​
​
​
​
​
​
Due to the energy conservation mentioned above, the lengths of these dashed lines do not change, while outside of the assisting objects frame a change in speed can still be achieved. The key difference between an increase in speed or a braking manoeuvre is the spacecraft passing behind or infront of the planet respectively. The magnitude of the final velocity of the planet from an outside reference frame is:
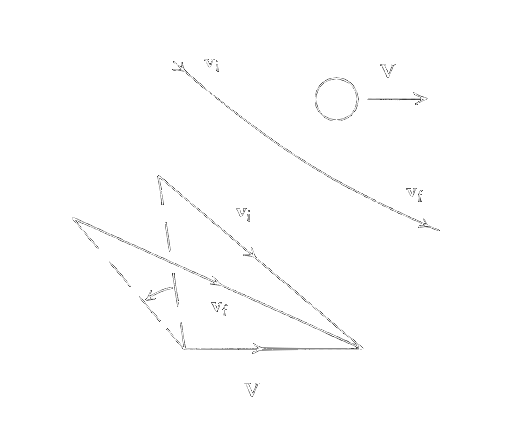
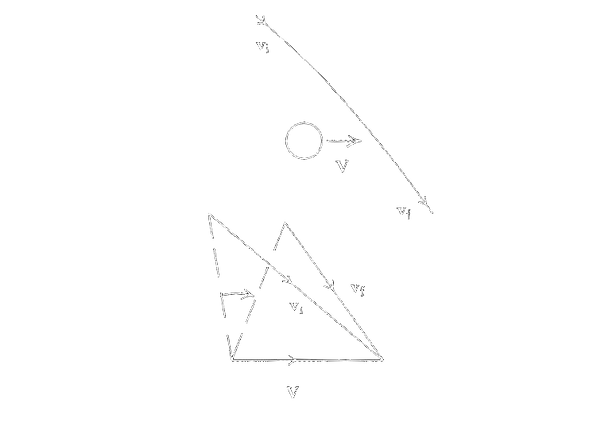

Where vi and vf are the distant-approach and distant-departure velocities outside of the planet’s reference frame, β is the deflection angle of the spacecraft in the planet frame and α is the angle between the positive direction of V and vi.
vf is equal to vi when β equals zero so the spacecraft experiences maximum boost when vf aligns with V.
With vi, V and α fixed vf increases with β, reaching its maximum at β = βmax where:

Interstellar
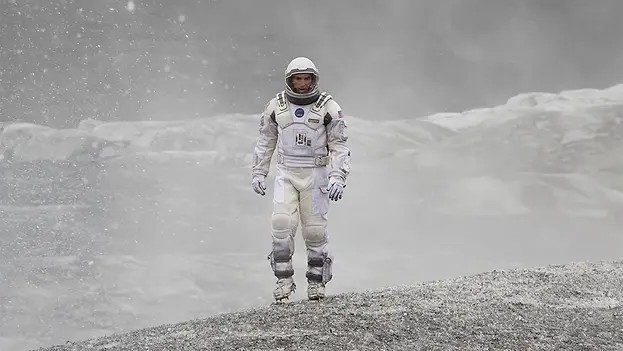
In Interstellar, the Endurance spacecraft realistically depicts artificial gravity via rotation, as discussed in the background theory. The spacecraft features a ring-shaped design and rotates at a set speed to simulate a gravity similar to Earth’s.
​
It is without a doubt that the artificial gravity portrayed in Interstellar is scientifically plausible and retains a good degree of scientific accuracy. However, the film does not accurately depict the challenges associated with the generated artificial gravity – it does not portray the nauseating effects of Coriolis force as the crew move towards and away from the centre of rotation and it also seemingly does not portray the small variations of gravity that the crew would experience in moving with or against rotation.
​
It is understandable that these details are omitted from the plot, though. It seems Christopher Nolan agreed that providing fanatics with a high-octane space movie was of more benefit than providing an emetophobic viewer with 3hrs of their greatest nightmares. With the cinematic experience of great importance, it is no surprise that details such as the Coriolis effect were omitted; disregarding these omissions, the film is highly accurate.
​
In Interstellar, the Endurance crew utilize multiple gravitational slingshot manoeuvres to adjust their speed and trajectory efficiently, whilst reducing fuel consumption. In many ways, Interstellar’s depiction of this is plausible and many gravity assists similar to the ones depicted in the movie have been performed in real space missions. There are some serious shortcomings in the movie, however.
​
Early in the movie, the crew uses the gravitational field of Mars to slingshot the Endurance spacecraft toward Saturn and its nearby wormhole. It is noted to take 8 months to travel to Mars, and a further 14 months from Mars to Saturn. This is a realistic depiction of the slingshot manoeuvre and conveys the core principles – the ship uses the gravity of Mars to increase their speed significantly, allowing the crew to traverse the significantly larger distance of Mars to Saturn in a time not much longer than that to Mars itself. This is similar to real-life uses of gravity assist. Although mars is a lot smaller than planets typically used before for gravity assists, its high velocity towards Saturn relative to the sun should still be enough for success. ​
Later in the movie, however, the Endurance crew find themselves low on fuel and see no option other than to perform another slingshot manoeuvre to reach their destination (Edmund’s planet). More dramatically this time, the slingshot is performed using the supermassive black hole, Gargantua, which had been looming over them for duration of the movie. Unlike the Mars manoeuvre, this is more unrealistic and significantly oversimplifies the physics involved. Namely, due to the sheer strength of Gargantua’s gravity, performing a manoeuvre as such would be almost impossible - the Endurance would be subject to
​
​
​
​
So, while Interstellar accurately depicts the art of slingshotting at certain points in the movie, such as that regarding Mars at the beginning, it severely oversimplifies the physics at play at other points. It goes without saying that Gargantua slingshot scene had us all on our seats, though. So perhaps we can forgive the producers for taking certain Hollywood liberties to elevate our viewing experience. A scene in which the crew were spaghettified during this manoeuvre would have been entertaining, without a doubt, but also a sure waste of such fantastic plot they had crafted up until this.
2001: A Space Odyssey

Artificial gravity is shown multiple times in the film. Early in the film a rotating ring-shaped space station is shown, the crew can walk on the inside of its ring shape curving around its centre of rotation. This satellite's large size allows passengers to live comfortably under the effects of artificial gravity. Artificial gravity is also shown later in the film the much smaller spacecraft Discovery 1. However, rather than the whole ship rotating its crew quarters are within a rotating centrifuge inside the ship. This allows the crew to walk around the walls on the inside of the centrifuge. In a rotating environment with a radius as small as the crew quarters, the artificial gravity would vary significantly at the crew’s feet, waist, and head. See our artificial gravity tool for an idea of how the perceived weight may change at different distances from the axis of rotation.
​
There are also other ways the crew adapt to weightlessness when not in a rotating spacecraft. The astronauts can walk upright in zero gravity using “grip shoes", which keep the wearer connected to various surfaces inside the ship using hook-and-loop fasteners. The actors’ choreography is done well, showing the awkward feeling that would come with walking using these shoes rather than having the artificial gravity present in the rest of the film.​
This scene shows the man walking through a connecting chamber that is seemingly still, compared to the rotating frame of the chamber he is entering. This makes sense, as the artificial gravity experienced in the final chamber would result from rotation. However, it does not make sense for him to have gravity in the connecting chamber that is seemingly still. Also, when climbing into the final chamber after exiting the connecting tunnel, he descends to the ground level of the final chamber via a ladder, away from the axis of rotation. In this event, the person would experience Coriolis force which would result in varying “gravitational forces” being applied to the different parts of the body and ears, likely resulting in nausea or dizziness which is not depicted in the movie.
​
​
​
​
​
​
In many other science fiction works, space travel is often depicted with much faster-than-light travel or artificial propulsion systems, such as warp drives, with very little explanation as to how they might function. Clarke chose a different path, focusing on realism and the limitations of human technology, however, Kubrick’s filming did take a few artistic liberties for the sake of the film’s plot. By depicting orbital mechanics and practical spaceflight techniques they emphasised the scale of space exploration, making 2001: A Space Odyssey one of the more scientifically grounded sci-fi works of its time.

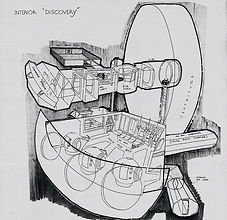
Exterior of Spaceship 'Discovery One' (top).
Interior plan schematic concept with the centrifuge from artist,
Shane Johnson (bottom).
Although not explicitly mentioned in Kubrick’s film, gravitational assists are directly described in Clarke’s novel. The spacecraft Discovery 1 uses a gravitational assist from Jupiter by entering its gravitational well to gain velocity towards one of its moons, without having to use extra fuel. This is depicted in the film so its safe to say they were following the ideas of Clarke’s novel for this without going too deep into the technical details.
​
Another scientific inaccuracy is a moment in the film where one of the crew survives the vacuum of space by holding his breath, in reality this would rupture his lungs. In an interview on the 2007 DVD release of the film, Clarke states that had he been on set for this scene, he would have changed this.
extreme tidal forces (variations in gravity), which it would not be able to withstand. It would be spaghettified (this is a real scientific phenomenon!); this would be unideal for McConaughey. In a hypothetical world, where the Endurance and its crew are not stretched into Fetuccine, the movie’s depiction also omits the complications that would arise from Gargantua’s own motion (it would spin, very fast), therefore breaking the law of conservation of momentum and disregarding trajectory complications for the Endurance. Interstellar depicts the Gargantua slingshot similarly to that of Mars earlier in the movie but these situations would greatly differ in reality.
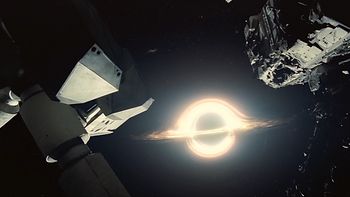
"We let Gargantua pull us down close to her horizon.
Then a powered slingshot around, launching us towards Edmunds' planet."
​
A quote from Mathew McConaughey's character, Cooper, in Interstellar.
We rate: "Interstellar"
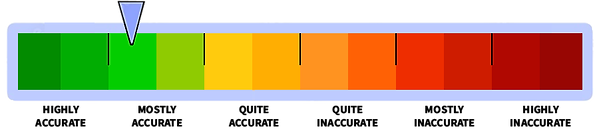
We rate: "2001: A Space Odyssey"

How do they compare?
Interstellar and 2001: A Space Odyssey both strive for scientific realism in their depictions but reflect the scientific understanding of their respective times. A Space Odyssey meticulously portrays space travel as it was envisioned in the 1960s, with realistic portrayals of artificial gravity and practical spaceflight techniques, aside from an absence of more recent, complex concepts, such as gravity assist manoeuvres. Its rotating space station demonstrates strong scientific accuracy, though omits certain physiological challenges that arise from artificial gravity, such as nausea due to the Coriolis effect or varying forces across the body.
​
Interstellar, benefitting from modern scientific advancements, presents a similarly realistic take on artificial gravity in its depiction of the Endurance spacecraft, though it also omits side effects such as the Coriolis effect. It expands upon A Space Odyssey’s realism by incorporating the concept of gravity-assist manoeuvres as a crucial technique for the Endurance’s traversal of space. It accurately depicts a manoeuvre using Mars in the early stages of the movie, though pushes the boundaries of science when later exploring the same manoeuvre using the black hole – something incredibly implausible due to the unfathomable strength of the gravity of black holes.
​
Both films retain a great amount of scientific accuracy but take certain concessions for improved engagement with the audience on the big screen. A Space Odyssey is grounded in real-world physics with few embellishments, whereas Interstellar makes greater use of creative license for shock factor. Nevertheless, both films exist as remarkable examples of scientifically accurate science-fiction media and portray the concept of artificial gravity in an accurate and extremely similar fashion. See below, a comparison of an early 50s NASA spacecraft concept aside the spacecraft from Interstellar and A Space Odyssey. Near identical!
NASA concept, 1952:
"Von Braun's space station"
Interstellar:
"Endurance"
2001: A Space Odyssey:
"Space Station V"
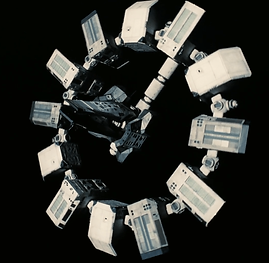


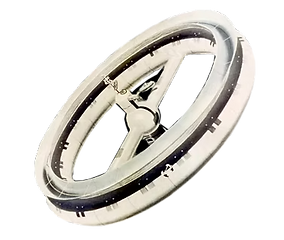
